Looking to the future of immunotherapy in cancer treatment
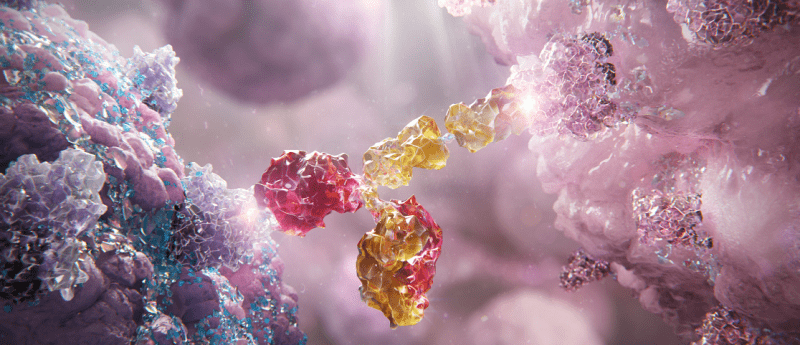
This article has been commissioned and funded by AstraZeneca
Millions of people with cancer already benefit from immunotherapy, but there is always more we can do to develop transformative treatments for patients in need. At AstraZeneca, we have developed a novel immuno-oncology research framework that articulates the role of the immune system in cancer. By putting our framework into practice, we are building a diverse pipeline focused on bringing emerging modalities, such as immune-cell engagers and next-generation cell therapies, to the broadest possible group of patients.
Introducing our immuno-oncology (IO) research framework
At AstraZeneca, we are taking a multifaceted approach to tackling cancer. Our diverse portfolio includes a range of mechanisms and modalities designed to either kill cancer cells directly, or to activate an anti-tumor immune response.
In the field of IO, we are exploring innovative ways to amplify the body’s natural defenses, by empowering the immune system to better detect and eliminate cancer while sparing healthy cells.
To guide our future approach in this space, we are adopting our innovative research framework that articulates the role of the immune system in recognizing and engaging with tumor cells.
The framework is based on our current understanding of the intricacies of the human immune system, as seen from a wider evolutionary perspective. Taking a wide view helps us to understand what is unique about human immunity and how it can be targeted in the most effective way, with the ultimate goal of delivering potentially transformative treatments to more people living with cancer.
What is the framework?
Our proposed model delineates the decision-making processes made by the immune system in response to oncogenic transformation, transiting among three distinct states, namely ‘ignore’, ‘defend’, and ‘attack’. Upon recognizing something as foreign or harmful, the immune system must first decide whether to enter attack or defend mode. In either state, immune cells can be disengaged (ignore), engaged and active (attack), or engaged but suppressed (defend). Our intention is to leverage this conceptual framework to expedite the discovery of innovative therapeutic interventions in oncology. The objective is to augment the immune system’s proficiency in identifying and eradicating cancer cells, effectuating a shift from the ‘ignore’ or ‘defend’ states towards the ‘attack’ state.
From ‘ignore’ to ‘attack’: recruit and engage
‘Ignore’ reflects a state where immunity is not engaged and the immune system is unable to recognize tumor cells. This may result from either a limited number of tumor antigens being present in the tumor, for example due to low mutation burden, the absence of T-cell receptors that can recognize tumor antigens, or from defects in the antigen presentation machinery.
To overcome this, we are designing therapies aimed at creating new or ‘synthetic immunity’ to drive immune recognition. For instance, this can be achieved through the genetic modification of T cells to create cell therapies (or ‘living medicines’) targeting specific tumors [1], or by engineering antibodies that create artificial immune synapses between tumor cells and T cells that are present in patients [2–4].
An additional mechanism whereby cancer cells are ‘ignored’ by the immune system involves the exclusion of immune cells from the tumor microenvironment (TME) [5,6]. This prevents T cells from entering the tumor, and we are exploring new ways to create a more immune-permissive TME, like using ATR inhibitors or targeted delivery of cytotoxic agents using antibody drug conjugates (ADCs).
These approaches could potentially restore the body’s ability to recruit and engage the immune system against cancer.
From ‘defend’ to ‘attack’: remove suppression and reinvigorate
The ‘defend’ state is where the immune system is engaged but restrained by immune tolerance mechanisms. For example, many tumors can evade attack by modulating immune checkpoints such as PD-L1 and TGFβ, resulting in the negative regulation of T cells [7,8]. Our aim is to empower T cell immunity by blocking inhibitory mechanisms or restoring T cell cytotoxic function.
We know that the presence of T cells in tumors is associated with response to existing immunotherapies [5], and we are continuously enriching our understanding of the role played by other immune cells, including myeloid-derived suppressor cells [6]. We are researching immune cell populations in the TME, as a deeper understanding of the immunological composition of a tumor is integral to discerning optimal therapeutic strategies [6,9–12].
By adopting a human immunity-centric approach to the ‘defend’ state, we anticipate gaining a better understanding of how to modulate the TME, with the goal of overcoming immune cell exhaustion and rendering tumors vulnerable to attack.
Putting the framework into practice
Despite considerable advancements in immunotherapy in oncology, with the emergence of checkpoint inhibitors and next-generation agents such as immune-cell engagers, there remains a need for innovative therapies for patients who do not respond to these treatments, or those who develop resistance [1,13]. To address these therapeutic gaps, at AstraZeneca we are exploring a range of immunotherapeutic strategies, including cell therapies, to expand the breadth of treatment options available for more people living with cancer.
In line with our IO research framework, we are advancing a variety of innovative mechanisms and treatment modalities that aim to alleviate immunosuppression and reinvigorate the immune system, through a shift from the ignore or defend state into attack.
Even in more treatment-sensitive tumor types, not every patient responds to immunotherapies, while even responders frequently relapse after treatment [13]. By building a diverse pipeline of immunotherapies, including cell therapies, we aim to expand treatment options available for more people living with cancer.
‘Ignore’ to ‘attack’ in practice:
Immune-cell engagers
Immune-cell engagers are gaining recognition as a promising therapeutic approach in the treatment of both hematologic and solid cancers [2]. These engineered antibodies are designed to forge an artificial immune synapse, bridging the gap between a tumor cell antigen and an immune cell triggering molecule, such as CD3 on T cells [2,3]. The formation of this synapse triggers T cell activation, precipitating direct tumor cell killing, while simultaneously shifting the immune system into an ‘attack’ state in the vicinity of cancer cells that may otherwise evade detection. Additionally, T cells in the proximity, despite being in an inactive state, could potentially be mobilized and activated by local ‘attack’ signals, steering their activity against the tumor, and inducing the recruitment of additional immune cells via cytokine release, thereby amplifying the immune response.
Next-generation cell therapies
We are also committed to driving the future of cell therapies in oncology. Autologous chimeric antigen receptor T cell (CAR-T) therapies, which involve engineering the patient’s T cells for cancer targeting, are among the most successful new treatment modalities in recent years [1,14]. These drugs have achieved their success, including cures in some cases, by inducing the immune system to enter an ‘attack’ state.
Since the first CAR-T approval in 2017, the field has been growing exponentially [1,15]. Replicating the success of CAR-T therapies in blood cancers, in solid tumors is a critical objective for our team at AstraZeneca. To do this, we need to help cell therapies penetrate solid tumor tissue.
Directing CAR-Ts against solid tumors with supplementary co-medication
It is well established that a hostile, suppressive TME hampers the infiltration and expansion of T cells, even when they have been engineered to target cancer cells [6,9].
Advances in our understanding of immune function in suppressive conditions are helping us develop new ways to engineer CAR-Ts, with the goal of improving their activity and achieving longer-lasting effective immunity in the TME. Starting with the inhibition of the anti-inflammatory signaling cytokine, transforming growth factor-β, we are developing a portfolio of molecules designed to support CAR-T therapies to combat and reverse immunosuppression.
T-cell receptor therapy (TCR-T): A complementary approach in cell therapy
Another emerging modality showing early promise is targeting TCR-Ts. The T-cell receptor (TCR) is an antigen receptor that recognizes peptide antigens bound to the major histocompatibility complex (MHC) on target cells, leading to the activation of T cells and stimulation of the immune system [16].
Through genetic engineering, scientists can use TCRs to edit the antigen-specificity of T cells to recognize specific targets including neoantigens that are derived from tumor-specific mutations [17]. By exploiting the cell’s natural antigen-recognition mechanism, TCR-Ts can identify a wide range of targets including intracellular proteins, unlocking new biology, and complementing CAR-Ts that recognize extracellular proteins [17,18]. AstraZeneca has recently acquired Neogene Therapeutics, a leader in the field of TCR-T development, complementing our efforts in CAR-Ts.
Off-the-shelf therapies
Looking to the future, our vision is to increase access to cell therapies by developing off-the-shelf, patient-ready CAR-Ts and TCR-Ts directed at common cancer targets, to overcome challenges of scalability and the time-consuming personalized manufacturing of other cell therapies [19].
The development of cell therapies continues to be a promising avenue for further investigation, fulfilling our framework-defined goal of targeting hard-to-treat tumors by switching the immune system from an ‘ignore’ to an ‘attack’ state.
‘Defend’ to ‘attack’ in practice:
Bispecific antibodies
Currently, we are investigating the potential for achieving therapeutic synergy through protein engineering to combine two separate antibodies as a single molecule, known as bispecific antibodies [20].
We are exploring the potential for bispecific antibodies to target common resistance mechanisms to current therapies and to target multiple inhibitory checkpoints simultaneously, with the goal of driving a stronger shift from “defend” to “attack.” With these capabilities, this modality could unlock new biology and potentially improve treatment outcomes for people living with cancer.
The future of IO
Cancer immunotherapy research has rapidly evolved over the past decade with the development of checkpoint inhibitors and the emerging promise of next-generation agents such as immune-cell engagers and cell therapies. However, there is still a need for more effective therapies for patients who do not respond to these treatments, or those who develop resistance.
In line with our IO research framework and strategy, we are researching a variety of innovative modalities to empower the immune system against cancer. By building a diverse pipeline of immunotherapies, including cell therapies, we have the opportunity to explore novel combinations in our broader oncology portfolio with the goal of driving stronger, more durable responses.
We are living in a golden age of cancer immunotherapy with an unprecedented rate of drug approvals for oncology. My hope is that over the next decade, using our strategic framework we can extend, expand, and improve upon this golden age to deliver the right medicines to patients in need, and ultimately transform what it means to be diagnosed with cancer.
Author profile:
Mark Cobbold – Vice President Discovery and Head of Oncology Cell Therapy, Oncology R&D, AstraZeneca
Find out more about out how we’re accelerating innovation to transform cancer care: Accelerating innovation in cancer treatment
Learn more about the tumour microenvironment and role of myeloid cells in the TME: The tumour microenvironment – Unlocking anti-tumour immunity
References:
- Saez-Ibañez AR, Upadhaya S, Partridge T, Shah M, Correa D, Campbell J. Landscape of cancer cell therapies: trends and real-world data. Nat. Rev. Drug Discov. 21(9):631–2 (2022).
- Fucà G, Spagnoletti A, Ambrosini M, de Braud F, Di Nicola M. Immune cell engagers in solid tumors: promises and challenges of the next generation immunotherapy. ESMO Open. 6(1)(2021).
- Huehls AM, Coupet TA, Sentman CL. Bispecific T-cell engagers for cancer immunotherapy. Immunol. Cell Biol. 93(3):290–6 (2015).
- Clinicaltrials.gov. A Study of TNB-486 in subjects with relapsed or refractory B-cell Non-Hodgkin Lymphoma. Available at: https://clinicaltrials.gov/ct2/show/NCT04594642. Accessed 27 June 2022.
- Maleki Vareki S. High and low mutational burden tumors versus immunologically hot and cold tumors and response to immune checkpoint inhibitors. J. Immunother. Cancer. 6(1) (2018).
- Barry ST, Gabrilovich DI, Sansom OJ, Campbell AD, Morton JP. Therapeutic targeting of tumour myeloid cells. Nat. Rev. Cancer 23:216–237 (2023).
- Seliger B. Basis of PD1/PD-L1 therapies. J. Clin. Med. 8(12) (2019).
- Yi M, Li T, Niu M, Wu Y, Zhao Z, Wu K. TGF-β: A novel predictor and target for anti-PD-1/PD-L1 therapy. Front. Immunol. 13 (2022).
- Anderson NM, Simon MC. The tumor microenvironment. Current Biology30:905–31 (2020).
- van Vlerken-Ysla L, Tyurina YY, Kagan VE, Gabrilovich DI. Functional states of myeloid cells in cancer. Cancer Cell 41(3):490–504 (2023).
- Kim R, Hashimoto A, Markosyan N et al. Ferroptosis of tumour neutrophils causes immune suppression in cancer. Nature 612(7939):338–46 (2022).
- Tcyganov EN, Sanseviero E, Marvel D et al. Peroxynitrite in the tumor microenvironment changes the profile of antigens allowing escape from cancer immunotherapy. Cancer Cell 40(10):1173-1189.e6 (2022).
- Bai R, Chen N, Li L, et al. Mechanisms of Cancer Resistance to Immunotherapy. Front Oncol. 10 (2020).
- Finck A V., Blanchard T, Roselle CP, Golinelli G, June CH. Engineered cellular immunotherapies in cancer and beyond. Nat. Med. 28(4):678–89 (2022).
- Kankeu Fonkoua LA, Sirpilla O, Sakemura R, Siegler EL, Kenderian SS. CAR T cell therapy and the tumor microenvironment: Current challenges and opportunities. Mol. Ther. Oncolytics. 25:69–77 (2022).
- Philipa Marrack, John Kappler. The T-cell Receptor. Science 238(4830):1073–9(1979).
- Shafer P, Kelly LM, Hoyos V. Cancer therapy with TCR-engineered t cells: current strategies, challenges, and prospects. Front. Immunol. 13 (2022).
- Zhao L, Cao YJ. Engineered T Cell therapy for cancer in the clinic. Front. Immunol. 10:2250 (2019).
- Cutmore LC, Marshall JF. Current perspectives on the use of off the shelf CAR-T/NK cells for the treatment of cancer. Cancers (Basel). 13(8) (2021).
- Dahlén E, Veitonmäki N, Norlén P. Bispecific antibodies in cancer immunotherapy. Ther. Adv. Vaccines Immunother. 6(1):3–17 (2018).
August 2023 | VeevaID Z4-57038
In association with:
The opinions expressed in this article are those of the author and do not necessarily reflect the views of Oncology Central or Future Science Group.